All published articles of this journal are available on ScienceDirect.
Adipose-derived Stem Cells Antagonize Fibrotic Response of Keloid-derived Fibroblasts
Abstract
Background
Keloid is an excessive fibrotic condition that results in excessive deposition of extracellular matrix (ECM), mainly composed of collagen. Transforming growth factor-β (TGF-β) is a cytokine involved in keloid development by stimulating ECM production and fibrosis. Mesenchymal stem cells (MSCs) are multipotent non-hematopoietic progenitor cells that regulate the immune response. Adipose-derived stem cells (ADSCs), which are MSCs, are present in the stromal portion of the adipose tissue and are accessible for clinical use.
Objective
This study aimed to investigate the effects of ADSCs on the fibrotic responses of keloid-derived fibroblasts.
Methods
Keloid-derived fibroblasts cultured in the presence of transforming growth factor-β-1 (TGF-β1) were co-cultured with ADSCs. Immunofluorescence microscopy, real-time polymerase chain reaction, and western blotting were performed to determine the expression levels of smooth muscle protein 22-α (SM22α), type I collagen (COL1), TGF-β1, matrix metallopeptidase 2 (MMP2), SMAD2, SMAD3, platelet-derived growth factor receptor α (PDGFRα), and TGF-β receptor type-1 (TGFβR1). Keloid-derived fibroblast-embedded collagen gel contraction assay was also performed.
Results
Keloid-derived fibroblasts express SM22α, COL1, TGF-β1, MMP2, SMAD2, SMAD3, PDGFRα, and TGFβR1. TGF-β1 increased their expression levels, whereas ADSCs significantly suppressed them. TGF-β1 induced the contraction of keloid-derived fibroblast-embedded collagen gel, whereas ADSCs significantly inhibited it.
Conclusion
ADSCs antagonize the fibrotic effects of TGF-β on keloid-derived fibroblasts and may be a therapeutic agent for keloids. ADSCs may also suppress keloid development during normal wound healing.
1. INTRODUCTION
Myofibroblasts produce an extracellular matrix (ECM) that modulates tissue resilience and strength during wound healing. However, altered ECM deposition can also lead to tissue dysfunction. Keloid is an excessive fibrotic condition that results in excess deposition of ECM, mainly composed of collagen [1], and is associated with itching, pain, impaired aesthetics, and periarticular contractures. Although keloids are caused by cutaneous injury and irritation, such as burns, acne, and surgery, their pathogenesis and disease mechanisms remain unclear. In addition, there is no single satisfactory treatment for keloids, although they are treated with various therapies, including intralesional or topical corticosteroids, radiotherapy, compression therapy, and surgical excision.
Transforming growth factor-β (TGF-β) is a cytokine involved in keloid development by stimulating ECM production and fibrosis [2]. Keloid-derived fibroblasts are uniquely sensitive to TGF-β, with increased expression of transforming growth factor-β-1 (TGF-β1), transforming growth factor-β-2, and TGF-β type I and II receptors, resulting in collagen production [3-8]. Dysregulation of TGF-β/SMAD signaling is thought to be a major factor in keloid formation [9, 10]. TGF-β is the most potent inducer of myofibroblast differentiation [11].
Mesenchymal stem cells (MSCs), play a key role in tissue healing and are multipotent non-hematopoietic progenitor cells that regulate immune responses. MSCs secrete immunomodulatory and anti-inflammatory factors [12-14] and have been used to treat acute graft-versus-host disease [15]. Thus, MSCs may be of potential use not only for wound repair but also for inflammatory diseases. Adipose-derived stem cells (ADSCs), which are MSCs, are present in the stromal portion of the adipose tissue and are accessible for clinical use. ADSCs also play an important role in wound healing. ADSCs and dermal fibroblasts are major sources of ECM. However, ADSCs, the same as other MSCs, have potent immunomodulatory effects.
It was previously reported that intralesional injection of ADSCs reduced hypertrophic scarring [16, 17], although the underlying mechanism was not fully understood. Herein, we investigated the effects of ADSCs on the fibrotic responses of keloid-derived fibroblasts.
2. MATERIALS AND METHODS
2.1. Cell Culture
After the patients provided informed consent, keloid-derived fibroblasts were prepared from fresh human keloid tissues obtained at the time of surgical excision. The cells were cultured in Dulbecco’s modified Eagle’s medium (DMEM) containing 10% fetal bovine serum (FBS) and 100 μg/mL penicillin-streptomycin (Sigma).
ADSCs were purchased from Lonza (Lonza Group AG, Basel, Switzerland) and cultured in an ADSC growth medium containing 10% FBS, L-glutamine, and GA1000. ADSCs at passages 2–6 and keloid-derived fibroblasts at passages 2–7 were used for all the experiments in this study.
Millicell hanging cell culture inserts with a pore size of 0.4 mm (Merck Millipore) were used for the co-culture experiment. Keloid-derived fibroblasts (5 × 104 cells) cultured in the presence of TGF-β1 (10 ng/mL) were seeded in 6-well plates and cultured in DMEM containing 10% FBS for 24 h, whereas ADSCs (5 × 104 cells) were seeded on inserts with ADSC medium in the co-culture system. After the upper chamber was removed from the co-culture system after 48 h of incubation, total RNA or protein was extracted from keloid-derived fibroblasts in the bottom chamber. Positive controls were stimulated with 10 ng/mL TGF-β1 to the keloid-derived fibroblasts and then seeded into a 6-well plate without a co-culture system.
2.2. Immunofluorescence Microscopy
Keloid-derived fibroblasts, fibroblasts cultured in the presence of TGF-β1, and fibroblasts cultured in the presence of TGF-β1 co-cultured with ADSCs were stained for smooth muscle protein 22-α (SM22α), type I collagen (COL1), TGF-β1, matrix metallopeptidase 2 (MMP2), SMAD2, SMAD3, and platelet-derived growth factor receptor α (PDGFRα). The cells were fixed with a 4% paraformaldehyde solution and pre-incubated with 0.1% Triton X-100 for 10 min before being incubated with 5% bovine serum albumin for 1 h. They were incubated with primary antibodies against SM22α (1:100; Santa Cruz Biotechnology, CA, USA), COL1 (1:1000; Proteintech, Tokyo, Japan), TGF-β1 (2 μg/mL; Abcam, Cambridge, MA, USA), MMP2 (1:1000; Abcam), SMAD2 (1:1000; Cell Signaling Technology, Danvers, MA, USA), SMAD3 (1:1000; Cell Signaling Technology), and PDGFRα (1:1000; Cell Signaling Technology). After extensive washing, the sections were incubated with Alexa488-conjugated goat anti-mouse antibody or Alexa488-conjugated goat anti-rabbit antibody (1:1000; Life Technologies) for 45 min at room temperature. The samples were washed and incubated with secondary antibody (1:1000; Life Technologies) for 45 min at room temperature in the dark, followed by three times washing in phosphate-buffered saline. Nuclei were counterstained with ProLong Diamond (Thermo Fisher Scientific), and the samples were visualized using an EVOS M5000 fluorescence microscope (Thermo Fisher Scientific)18.
2.3. Real-time Polymerase Chain Reaction
The expression of SM22α, COL1, TGF-β1, MMP2, SMAD2, SMAD3, PDGFRα, and TGF-β receptor type-1 (TGFβR1) was evaluated using real-time polymerase chain reaction (PCR). Total RNA was extracted from keloid-derived fibroblasts using the RNeasy Plus Micro kit (QIAGEN, Hilden, Germany), according to the manufacturer’s protocol. RNA (2 μg) was converted to cDNA using the ReverTra Ace qPCR RT kit (Toyobo, Osaka, Japan). TaqMan Master Mix (Applied Biosystems, Foster City, CA, USA) was used to amplify 1 μg of cDNA for 50 cycles using a StepOnePlus system (Applied Biosystems) [18]. The expression of SM22α, COL1, TGF-β1, MMP2, SMAD2, SMAD3, PDGFRα, and TGFβR1 (using primers SM22a (TAGLN TaqMan probe Hs01038777_g1), COL1 (TaqMan probe Hs00164004_m1), TGF-β1 (TaqMan probe Hs00998133_m1), MMP2 (TaqMan probe Hs1548727_m1), SMAD2 (TaqMan probe Hs00998188_ mH), SMAD3 (TaqMan probe Hs00969210_m1), PDGFRα (TaqMan probe Hs00998018_m1), and TGFBR1 (TaqMan probe Hs00610320_m1), respectively; Applied Biosystems) was normalized to glyceraldehyde-3-phosphate dehydro- genase (GAPDH) levels, and relative mRNA levels was calculated by the comparative cycle threshold (Ct) method using the formula 2-ΔΔCt.
2.4. Western Blotting
The expression of SM22α, COL1, TGF-β1, MMP2, SMAD2, SMAD3, PDGFRα, and TGFβR1 was assessed using western blotting. The cells were harvested and lysed with M-PER Mammalian Protein Extraction Reagent (Thermo Fisher Scientific), a protease inhibitor cocktail (Sigma-Aldrich). The protein levels were measured using the Bradford method and were adjusted to 100 μg of protein.
The protein samples were separated by 10% Tris-glycine sodium dodecyl sulfate–polyacrylamide gel electrophoresis (Bio-Rad, Hercules, CA, USA) and transferred to a nitrocellulose membrane under denaturing conditions. After being blocked with 5% skim milk in Tris-buffered saline, the membranes were incubated with primary antibodies against SM22α (1:100; Santa Cruz Biotechnology), COL1 (1:1000; Proteintech), TGF-β1 (2 μg/mL; Abcam), MMP2 (1:1000; Abcam), SMAD2 (1:1000; Cell Signaling Technology), SMAD3 (1:1000; Cell Signaling Technology), PDGFRα (1:1000; Cell Signaling Technology), and TGFβR1 (1:100; Santa Cruz Biotechnology) overnight at 4 °C. The blots were probed for GAPDH using a monoclonal antibody (1:2000; BioLegend, San Diego, CA, USA) as a loading control. The membrane was then washed and incubated with anti-mouse or anti-rabbit peroxidase-conjugated secondary antibody (1:1000; Cell Signaling Technology) at room temperature for 45 min and then with SuperSignal West Dura Extended Duration Substrate (Thermo Fisher Scientific)18. The bands were visualized and quantified using multi-gauge software (Fujifilm, Tokyo, Japan).
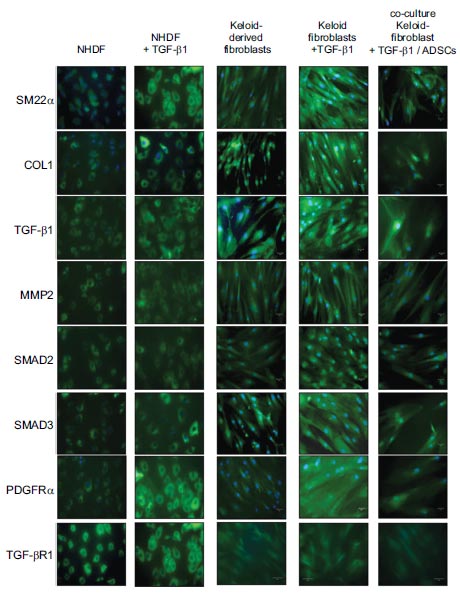
Keloid-derived fibroblasts express SM22α, COL1, TGF-β1, MMP2, SMAD2, SMAD3, and PDGFRα. TGFβ1 10 ng/mL increased the expression of these genes in keloid-derived fibroblasts, whereas co-culture with ADSCs significantly suppressed this effect. NHDF, normal human dermal fibroblasts; ADSCs, adipose-derived stem cells; COL1, type 1 collagen; MMP2, matrix metalloproteinase-2; PDGFRα, platelet-derived growth factor receptor α; SM22α, smooth muscle protein 22-α; TGF-β1, transforming growth factor-β1.
2.5. Collagen Gel Contraction Assay
The effect of ADSCs on keloid-derived fibroblast contraction in collagen gels was also examined [19-21]. Collagen solution was prepared by mixing acid-soluble porcine type I collagen (3 mg/mL), a 5-fold concentration of DMEM, and a buffer solution (0.05 M NaOH, 2.2% NaHCO3, 299 mM HEPES) in a 7:2:1 ratio (Nitta Gelatin Corp., Osaka, Japan).One mL of a mixture of keloid-derived fibroblasts (2.0 × 105 cells) suspension in serum-free DMEM and collagen solution was poured into each well of a 12-well plate and incubated at 37 °C for 30 min. After the gel solidified, 1 mL of serum-free DMEM was poured onto the gel to avoid dehydration. After incubation for 24 h, the gel was separated from each well and allowed to float. After 72 h, the surface area of each gel sample was measured.
3. RESULTS
The immunofluorescence analysis revealed that keloid-derived fibroblasts expressed SM22α. The addition of 10 ng/mL of TGF-β1 increased the expression of SM22α, which was significantly suppressed by co-culture with ADSCs (Fig. 1). Keloid-derived fibroblasts also express COL1, which is not produced by normal dermal fibroblasts without TGF-β1 [22]. The expression of COL1 was increased by TGF-β1 (10 ng/mL). However, co-culture with ADSCs significantly suppressed this effect (Fig. 1). The expression of TGF-β1, MMP2, SMAD2, SMAD3, and PDGFRα in keloid-derived fibroblasts was also increased by the addition of TGFβ1 (10 ng/mL). In contrast, co-culture with ADSCs significantly suppressed them (Fig. 1).
Real-time PCR revealed that TGF-β1 enhanced the expression of SM22α, COL1, TGF-β1, MMP2, SMAD2, SMAD3, PDGFRα, and TGFβR1 in keloid-derived fibroblasts, whereas co-culturing with ADSCs significantly suppressed them (Fig. 2).
Similar to the results of real-time PCR, western blotting showed that TGF-β1 enhanced the expression of SM22α, COL1, TGF-β1, MMP2, SMAD2, SMAD3, PDGFRα, and TGFβR1 in keloid-derived fibroblasts, whereas co-culture with ADSCs suppressed them (Fig. 3). These results suggested that TGFβR1 suppressed SM22α, COL1, MMP2, SMAD2, and SMAD3.
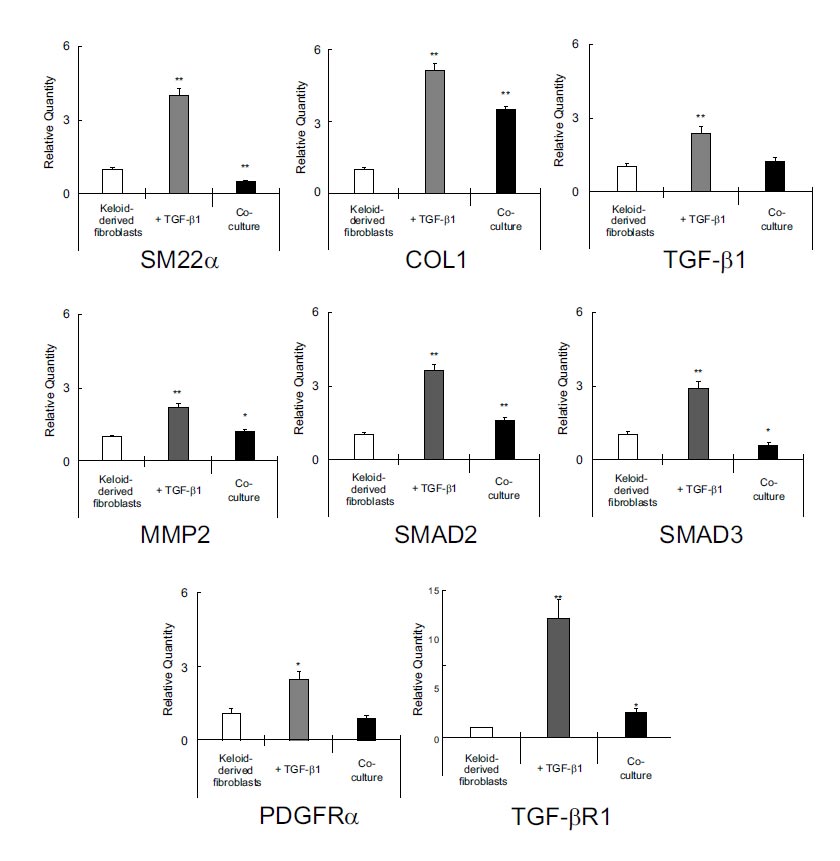
Real-time polymerase chain reaction revealed that TGF-β1 induced the expressions of SM22α, COL1, TGF-β1, MMP2, SMAD2, SMAD3, and TGFβR1 in keloid-derived fibroblasts, whereas co-culturing with ADSCs significantly suppressed them. ADSCs, adipose-derived stem cells; COL1, type 1 collagen; MMP2, matrix metalloproteinase-2; PDGFRα, platelet-derived growth factor α; SM22α, smooth muscle protein 22-α; TGF-β1, transforming growth factor-β1; TGFβR1, TGF-β receptor type-1.
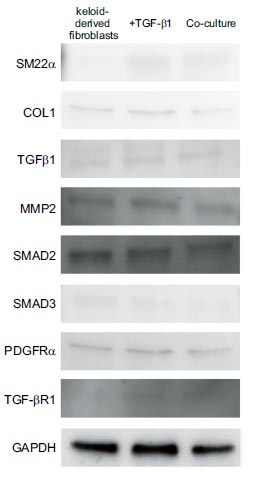
Similar to the results of real-time polymerase chain reaction, western blotting showed that TGF-β1 induced the expressions of SM22α, COL1, TGF-β1, MMP2, SMAD2, SMAD3, PDGFRα, and TGFβR1 in keloid-derived fibroblasts, whereas co-culturing with ADSCs suppressed them. COL1, type 1 collagen; MMP2, matrix metalloproteinase-2; SM22α, smooth muscle protein 22-α; TGF-β1, transforming growth factor-β1; TGFβR1, TGF-β receptor type-1.
The effect of ADSCs on the contraction of keloid-derived fibroblast-embedded collagen gels was examined. This assay investigates the contractile activity of cells, including fibroblasts [21], and TGF-β1 significantly induces collagen gel contraction [23]. At 72 h, keloid-derived fibroblasts showed a contraction of 69.4 ± 11.42% of the initial area without TGF-β1 (Fig. 4). TGF-β1 (10 ng/mL) enhanced the collagen gel contraction to 16.0 ± 8.48% of the initial area, whereas co-culture with ADSCs significantly inhibited the enhance of the collagen gel contraction to 32.1 ± 11.39%.
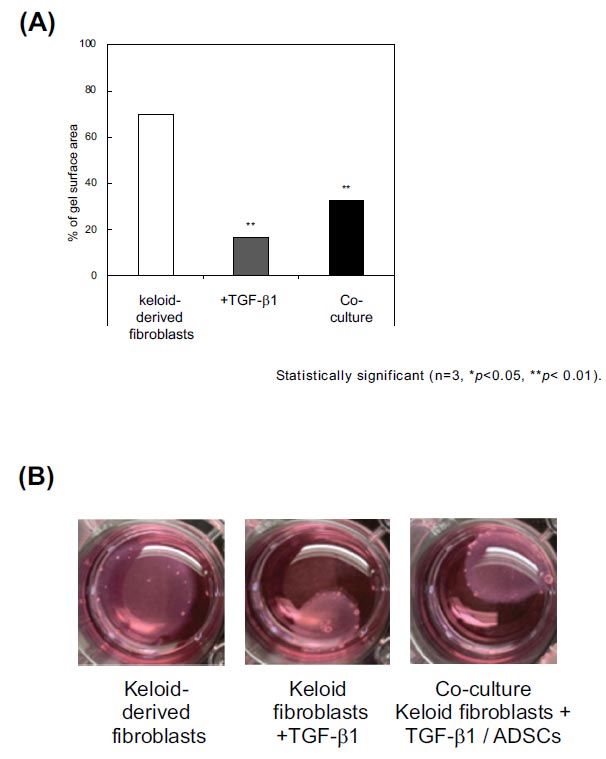
We examined the effect of ADSCs on the contraction of keloid-derived fibroblast-embedded collagen gel. TGF-β1 significantly induced contraction. At 72 h, keloid-derived fibroblasts showed a contraction of 69.4 ± 11.42% of the initial area without the addition of TGF-β1. TGF-β1 10 ng/mL induced contraction of the collagen gel to 16.0 ± 8.48% of the initial area, whereas co-culturing with ADSCs significantly inhibited it (32.1 ± 11.39%). ADSCs, adipose-derived stem cells; TGF-β1, transforming growth factor-β1.
4. DISCUSSION
The wound-healing process consists of inflammation, proliferation, and remodeling [24]. After skin injury, neutrophils and macrophages start inflammation and fibrotic processes by secreting growth factors, such as TGF-β and platelet-derived growth factor (PDGF). During the proliferation phase, granulation tissue forms to provide a structure for vascular growth, keratinocyte migration, and proliferation. In addition, wound contraction is mediated by myofibroblasts [25]. During the remodeling phase, the ECM is reorganized and degraded by various proteolytic enzymes, including matrix metalloproteinases (MMPs) and their inhibitors and tissue inhibitors of MMPs. As a result, the ratio of COL1 to type III collagen (COL3) increases [26], whereas the number of myofibroblasts decreases via apoptosis [27].
Inflammation is essential, although the critical processes involved in keloid development still need to be elucidated [25, 28]. Fibrogenic growth factors such as TGF-β, PDGF, fibroblast growth factor-β, and insulin-like growth factor I affect and transform fibroblasts into an enhanced scarring phenotype, α-Smooth muscle actin–positive myofibroblasts in keloids [7, 29]. Keloid-derived fibroblasts are characterized by increased expression of smooth muscle cell markers, COL1, MMP2, and contractile activity [21, 30]. They are highly sensitive to TGF-β1 and express higher collagen levels and other ECM-associated proteins than normal dermal fibroblasts4, and are thought to have a potential role in keloid development [22]. The differentiation of fibroblasts into myofibroblasts by TGF-β is critical for the development of human fibrotic disorder [31]. TGF-β mediates the SMAD signal transduction pathway via TGFβR1. In addition, keloid-derived fibroblasts have an enhanced response to platelet-derived growth factor (PDGF), which is mediated by elevated levels of PDGFRα [32]. SM-22α is expressed in smooth muscle cells and maintains the smooth muscle cell phenotype. Thus, we examined the effects of ADSCs on the expression of SM22α, a smooth muscle cell marker, COL1, TGF-β1, MMP2, SMAD2, SMAD3, PDGFRα, and TGFβR1, and a collagen gel contraction assay to determine whether ADSCs antagonized the fibrotic response in keloid-derived fibroblasts.
TGF-β1 is a cytokine with multiple functions, including cell proliferation and differentiation, and is secreted by fibroblasts, platelets, and inflammatory cells, particularly macrophages. In the wound healing process, TGF-β plays an important role in inflammation, angiogenesis, cell proliferation, collagen and ECM production, and wound remodeling. During the inflammation phase, TGF-β affects macrophages, enhances smooth muscle cell and fibroblast chemotaxis, and simultaneously regulates collagen and collagenase expression via the SMAD signaling pathway. Exogenous TGF-β is an important regulator of keloid formation because it is activated and upregulated in keloids and stimulates keloid fibroblast proliferation and collagen synthesis. In addition, inhibition of TGF-β signaling proteins reduces scar formation [33]. In our study, keloid-derived fibroblasts highly expressed TGF-β1, which enhanced the expression of SM22α, COL1, and TGF-β1.
It was previously reported that the production of TGF-β and collagen in keloid fibroblasts was suppressed by ADSCs over time compared with those in normal fibroblasts, consistent with our results [34]. In this study, a collagen sponge seeded with keloid fibroblasts was implanted in immunodeficient mice. Locally, injection of ADSCs significantly reduced the intensity of TGF-β and collagen production in keloid fibroblasts [34].
Proteolysis of ECM is necessary for wound remodeling. MMPs are essential for the degradation of excess ECM components, specifically COL1 and COL3 [35]. In keloids, the activity of these proteolytic enzymes is increased, which expands the area of wound remodeling [36]. MMP2 expression is particularly elevated in keloid-derived fibroblasts. The synthesis of proteases and MMPs is a highly complex process primarily regulated by TGF-β1 and SMAD [30]. Our results showed that keloid-derived fibroblasts highly expressed MMP2, a process enhanced by TGF-β1.
SMADs are a family of intracellular regulatory proteins classified as receptor-activated SMADs (SMAD1, 2, 3, 5, and 8), common mediator SMAD (SMAD4), and inhibitory SMADs (SMAD6 and 7) [37]. After phosphorylation, SMAD3 and SMAD4 form a complex [38]. In keloids, SMAD3 phosphorylation is upregulated, whereas SMAD3 downregulation significantly reduces procollagen gene expression in keloid fibroblasts [39]. Keloid formation is known to be suppressed by the inhibition of the TGF-β1-SMAD signaling pathway and Toll-like receptor 7 or SMAD7 activation [40]. Our study showed that ADSCs suppressed TGF-β1-enhanced TGFβR1, SMAD2, and SMAD3 expressions.
MSCs have immunomodulatory, antifibrotic, and angiogenic effects via paracrine growth factor secretion or cell-to-cell contact, as well as the ability to regenerate and transdifferentiate into various cell lineages [41-43]. MSCs administration modulates excessive inflammation, as demonstrated in experimental fibrotic disease models, such as pulmonary fibrosis, myocardial infarction, corneal injury, renal fibrosis, and liver cirrhosis [43-48]. MSCs are derived from the bone marrow and other tissues throughout the body. Among MSCs, ADSCs are relatively easy to harvest and can be obtained during resection surgery or liposuction.
CONCLUSION
In this study, we sought to determine whether ADSCs antagonize the TGF-β-mediated fibrotic response in keloid-derived fibroblasts. Through TGFβR1 downregulation, ADSCs inhibited TGF-β-induced COL1 expression, SM-22α expression, and contractile activity in keloid-derived fibroblasts. In addition, ADSCs suppressed the TGF-β-induced expression of MMP2 and PDGFRα. Thus, we concluded that ADSCs antagonize the fibrotic effects of TGF-β on keloid-derived fibroblasts and could be a therapeutic agent for keloids. ADSCs may also suppress keloid development during normal wound healing.
Although further investigation in animal experiments is needed for the clinical application of ADSCs to keloid treatment, there are no suitable animal models of keloids. Therefore, it is necessary to further accumulate the effects of ADSCs on keloids using various indicators in in vitro experiments.
AUTHORS’ CONTRIBUTION
It is hereby acknowledged that all authors have accepted responsibility for the manuscript's content and consented to itssubmission. They have meticulously reviewed all results and unanimously approved the final version of the manuscript.
LIST OF ABBREVIATIONS
ECM | = Extracellular matrix |
TGF-β | = Transforming growth factor-β |
TGF-β1 | = Transforming growth factor-β-1 |
MSC | = Mesenchymal stem cells |
ADSC | = Adipose-derived stem cell |
DMEM | = Dulbecco’s modified Eagle’s medium |
FBS | = Fetal bovine serum |
SM22α | = Smooth muscle protein 22-α |
COL1 | = Type I collagen |
MMP2 | = Matrix metallopeptidase 2 |
PDGFRα | = Platelet-derived growth factor receptor α |
TGFβR1 | = TGF-β receptor type-1 |
PCR | = Polymerase chain reaction |
GAPDH | = Glyceraldehyde-3-phosphate dehydrogenase |
Ct | = Cycle threshold |
PDGF | = Platelet-derived growth factor |
MMP | = Matrix metalloproteinase |
COL3 | = Type III collagen, NHDF, normal human dermal fibroblasts |
ETHICS APPROVAL AND CONSENT TO PARTICIPATE
The study protocol was approved by the Ethics Committee of Juntendo University Hospital (H21-0044).
HUMAN AND ANIMAL RIGHTS
No animals were used in this research. All procedures performed in studies involving human participants were in accordance with the ethical standards of institutional and/or research committee and with the 1975 Declaration of Helsinki, as revised in 2013.
AVAILABILITY OF DATA AND MATERIALS
The data supporting the findings of this study are available from the corresponding author [T.H] upon reasonable request.